Direct polymerization of functional monomers
Advantages:
-
Direct incorporation of functional groups into the polymer backbone
-
No post-polymerization modification required
-
High degree of functionality (DPfunctional monomer)
-
Arrangement dependent on (co)polymer architecture
-
Plethora of monomers with different functionality available
A number of monomers containing polar functional groups have been successfully polymerized by ATRP. They include acrylonitrile (AN),(1-5) (meth)acrylamides,(6,7) 4-vinyl pyridine (4VP),(8,9) dimethylaminoethyl methacrylate (DMAEMA),(10) and monomers containing an -OH group such as 2-hydroxyethyl acrylate (HEA)(11) and 2-hydroxyethyl methacrylate (HEMA).(12) Glycidyl acrylate(13) has also been polymerized by ATRP yielding well-defined polymers containing the reactive glycidyl group, which can be used as a precursor for other functional groups.(14) Water-soluble monomers (both neutral and ionic) can be polymerized in controlled fashion by ATRP directly in protic (aqueous) media.(15,16) Recently, an extension of the work focused on understanding the fundamentals of aqueous ATRP and defining conditions for better control, (17) allowed conditions for the ATRP of acidic monomers to be developed. (18) The knowledge that catalyst complexes remained stable under acidic conditions provided an incentive to investigate the reason for early termination of ATRP of acidic monomers. It was determined that the reason was the occurrence of an intramolecular cyclization reaction.
Three approaches were examined to overcome this problem which together allowed a significant increase in conversion and improved control over polymer growth: use of -Cl as the chain end halogen, lowering the pH (to 0.9), and increasing the polymerization rate. Methacrylic acid was polymerized by SARA ATRP and electrochemically mediated ATRP up to high conversion (90-99%), in t ≤ 4 h at ambient temperature, using inexpensive and relatively non-toxic reagents (NaCl, HCl, water) to control the concentration of chloride ions and pH of the aqueous polymerization medium. Control over molecular weight dispersity was satisfactory and molecular weights were in agreement with theoretical values. For example, a linear poly(methacrylic acid) with a degree of polymerization > 1000 and dispersity of 1.3 was synthesized in 3 h. An extension of the scope of catalysts suitable for direct polymerization of acidic monomers was exemplification of an iron porphyrin catalyst, derived from the active center of proteins such as horseradish peroxidase and hemoglobin, which was successfully used for the atom transfer radical polymerizations (ATRP) of methacrylic acid. ATRP of methacrylic acid and other acidic monomers. A robust mesohemin-based catalyst provided controlled ATRP of methacrylic acid, yielding poly(methacrylic acid) with Mn ≥ 20000 and dispersity Đ < 1.5. Retention of halogen chain end was confirmed by successful chain extension of a poly(methacrylic acid)–Br macroinitiator.(19)
Some examples of monomers, including some with polar groups that have been polymerized by ATRP, are shown below.
ATRP catalysts with strongly binding ligands should be used for copolymerization of monomers containing functional groups (mostly substituted amides, amines, or pyridines) to avoid, or reduce, competitive complex formation between the monomer or polymer and the copper center.(20) In many cases, catalyst destabilization can be suppressed by selection of the proper ligand or addition of excess ligand or excess "pseudo" ligand. For example, in the ATRP of sodium 4-styrenesulfonate in aqueous solution, there is a rapid disproportionation of the ATRP catalyst, resulting in loss of control. However, this disproportionation reaction is prevented in the presence of excess pyridine (a "pseudo" bpy ligand) or added bromide ions.(21) This is shown in the following table where water and a 1:1 water/pyridine mixture was used as the solvent for an ATRP polymerization of sodium 4-styrenesulfonate with a CuBr/bpy catalyst using a MePEOBiB initiator at 100:1 ratio at 30 0C.
The reaction was clearly controlled to a greater degree in the presence of a large molar excess of pyridine, which functions as a "pseudo" ligand, to ensure that the CuBr/bpy catalyst complex remains in solution.
While the ATRP of several types of polar monomers, particularly acidic ones, has proven to be quite challenging, progress continues to be made.(20, 22, 23)
Other Functional Monomers Successfully Polymerized by ATRP
The following schematic shows some of the monomers polymerized by ATRP and include styrenes, (meth)acrylates, (meth)acrylamides. While polymerization of monomers with free acidic groups were only recently polymerized in a controlled manner, see above section, monomers with other useful functionalities can be incorporated into a copolymer if attention is given to presevation of an active catalysy complex throughout the reaction since functionality often dictates the appropriate conditions for an ATRP, solvent, temp, catalyst, etc.. The use of functional macroinitiators allow incorporation functional monomer units that are generally polymerized by different mechanisms.Ionic Liquid Monomers
In order to evaluate the potential for poly(ionic liquid)s in applications a new class of 1-functionalized-3-methyl triazolium Tf2N- ionic liquid monomers were prepared and polymerized by ATRP. (25, 26)
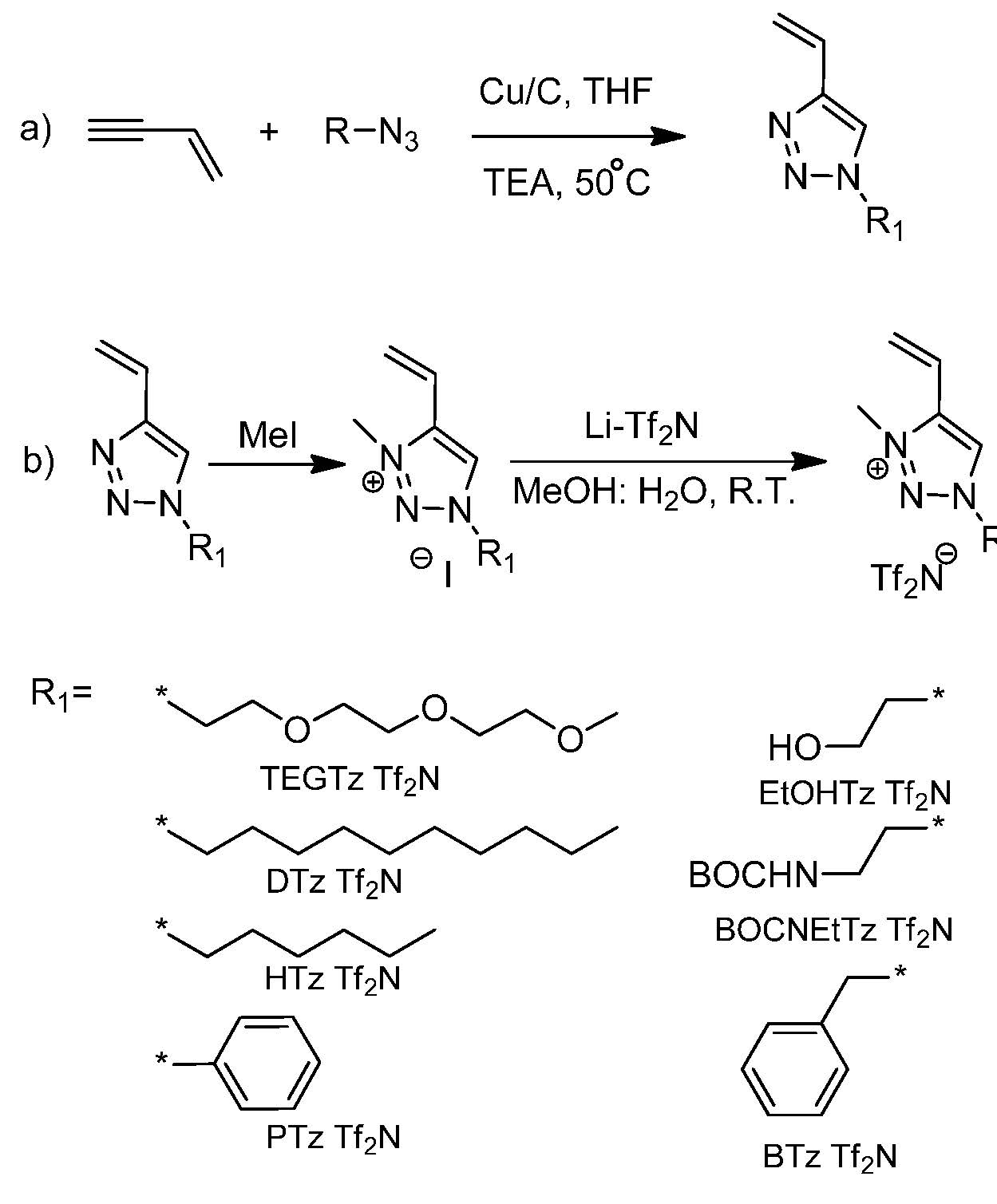
The use of vinyl acetylene, in the synthesis scheme shown above, provided access to a wide range of PIL monomers suitable for ATRP in a single step. The properties of the resulting PILs with pendant functional groups that influence the solubility, Tg and thermal stability provided access to specific properties for targeted applications.
The influence of the salt/counterion on the ATRP of the ionic liquid monomers was systematically examined in a later paper. (26) Removal of trace amounts of coordinating chloride ion from the pristine ionic liquid monomer prior to polymerization allowed a controlled ATRP to be conducted with a ratio of copper to initiator of 1:1 providing polymers with narrow MWD, ~1.1-1.2.
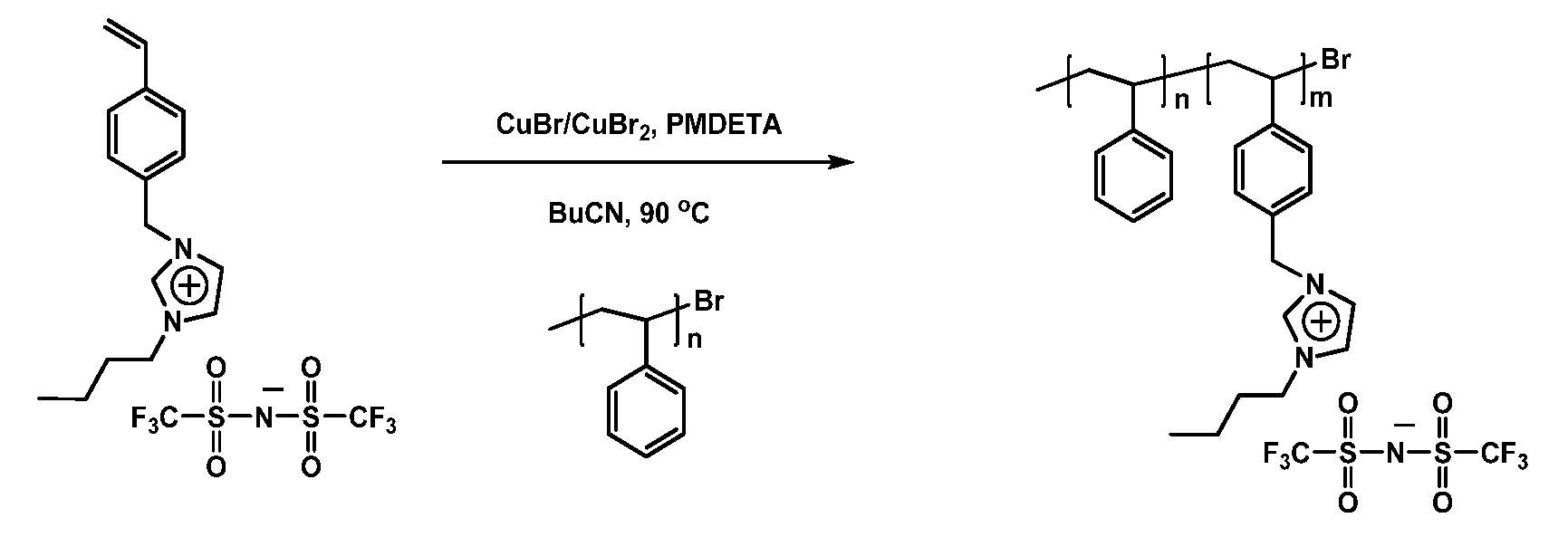
Modular polymerized ionic liquid block copolymer membranes, prepared by RAFT polymerization and a thio-Michael reaction to incorporate the ionic functionality, were evaluated for CO2/N2 separation. (25) When the composition of the PIL block copolymers were selected so that the bulk copolymers underwent phase separation, these materials show increased permeability relative to PILs while maintaining CO2/N2 selectivity, suggesting that PIL-containing BCPs, prepared by the more direct ATRP procedure outlined above, could be useful materials for the economical separation of CO2 from flue gas emissions.
REFERENCES
(1) Matyjaszewski, K.; Jo, S. M.; Paik, H.-j.; Gaynor, S. G. Macromolecules 1997, 30, 6398-6400.
(2) Matyjaszewski, K.; Jo, S. M.; Paik, H.-j.; Shipp, D. A. Macromolecules 1999, 32, 6431-6438.
(3) Tsarevsky, N. V.; Sarbu, T.; Goebelt, B.; Matyjaszewski, K. Macromolecules 2002, 35, 6142-6148.
(4) Kowalewski, T.; Tsarevsky, N. V.; Matyjaszewski, K. Journal of the American Chemical Society 2002, 124, 10632-10633.
(5) Tsarevsky, N. V.; Bernaerts, K. V.; Dufour, B.; Du Prez, F. E.; Matyjaszewski, K. Macromolecules 2004, 37, 9308-9313.
(6) Teodorescu, M.; Matyjaszewski, K. Macromolecules 1999, 32, 4826-4831.
(7) Teodorescu, M.; Matyjaszewski, K. Macromol. Rapid Commun. 2000, 21, 190-194.
(8) Xia, J.; Zhang, X.; Matyjaszewski, K. Macromolecules 1999, 32, 3531-3533.
(9) Tsarevsky, N. V.; Braunecker, W. A.; Brooks, S. J.; Matyjaszewski, K. Macromolecules 2006, 39, 6817-6824.
(10) Zhang, X.; Xia, J.; Matyjaszewski, K. Macromolecules 1998, 31, 5167-5169.
(11) Coca, S.; Jasieczek, C. B.; Beers, K. L.; Matyjaszewski, K. J. Polym. Sci., Part A: Polym. Chem. 1998, 36, 1417-1424.
(12) Beers, K. L.; Boo, S.; Gaynor, S. G.; Matyjaszewski, K. Macromolecules 1999, 32, 5772-5776.
(13) Matyjaszewski, K.; Coca, S.; Jasieczek, C. B. Macromol. Chem. Phys. 1997, 198, 4011-4017.
(14) Tsarevsky, N. V.; Bencherif, S. A.; Matyjaszewski, K. Macromolecules 2007, 40, 4439-4445.
(15) Matyjaszewski, K.; Tsarevsky, N. In PCT Int. Appl.; (Carnegie Mellon University, USA). WO 0228913, 2002; p 64 pp.
(16) Tsarevsky, N. V.; Matyjaszewski, K. Chemical Reviews 2007, 107, 2270-2299.
(17) Tang, W.; Kwak, Y.; Braunecker, W.; Tsarevsky, N. V.; Coote, M. L.; Matyjaszewski, K. J. Am. Chem. Soc. 2008, 130, 10702-10713.
(18) Tsarevsky, N. V.; Pintauer, T.; Matyjaszewski, K. Macromolecules 2004, 37, 9768-9778.
(20) Zhang, X.; Xia, J.; Matyjaszewski, K. Polym. Prepr. 1999, 40, 440-441.
(21) Huang, J.; Matyjaszewski, K. Macromolecules 2005, 38, 3577-3583.
(22) He, H.; Zhong, M.; Adzima, B.; Luebke, D.; Nulwala, H.; Matyjaszewski, K. J. Am. Chem. Soc. 2013, 135, 4227-4230.
(23) Adzima, B. J.; Taylor, S. C.; He, H.; Luebke, D. R.; Matyjaszewski, K.; Nulwala, H. B. J. Polym. Sci., Part A Polym. Chem. 2014, 52, 417-423.
(24) He, H.; Zhong, M.; Luebke, D.; Nulwala, H.; Matyjaszewski, K. J. Polym. Sci., Part A Polym. Chem. 2014, 52, 2175-84.
(25) Adzima, B. J.; Venna, S. R.; Klara, S. S.; He, H.; Zhong, M.; Luebke, D. R.; Mauter, M. S.; Matyjaszewski, K.; Nulwala, H. B. Journal of Materials Chemistry A: Materials for Energy and Sustainability 2014, 2, 7967-7972.