Theoretical Physics
Overview
One of the most remarkable aspects of nature is that it presents us with seemingly infinite variety of interesting phenomena, with characteristic length, time, and energy scales ranging over 60 orders of magnitude. The research activities of theoretical physicists at CMU span this entire range, and seek to address some of the most challenging open problems in particle physics, condensed matter physics, biological physics, gravitation and cosmology.
Many interesting questions in theoretical physics often arise when the interactions among the constituents of a physical system generate novel, emergent phenomena. How do quarks and their strong interactions determine the properties of protons, neutrons, and other hadrons? How do the components of biological systems influence their collective dynamic and mechanical properties? How do the thermal, electronic, and mechanical properties of condensed matter systems arise from the properties of the atoms they are made of, and the way these atoms are arranged? What are the properties of gravitational radiation emitted when two black holes form a binary inspiral? How does the matter and energy content of the universe give rise to the rich large scale structure we observe in our universe? These are only some of the questions that the theory group at CMU tackles using statistical mechanics, quantum mechanics, (quantum) field theory, and a variety of analytic and computational methods.
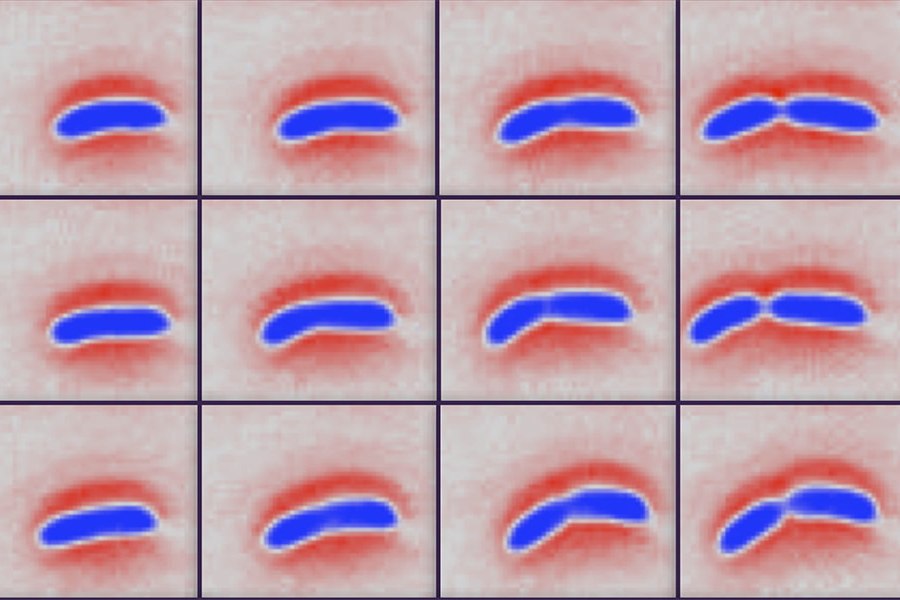
Shila Banerjee's group integrates theory, simulations, and data-driven modelling to understand how physical forces and chemical signalling control the shapes and architecture of living cells. They use concepts from statistical physics and dynamical systems to understand the principles that guide the organization and mechanics of sub-cellular matter, and predict how changes in sub-cellular organization control the spatiotemporal patterns of growth and movement in cells and tissues.
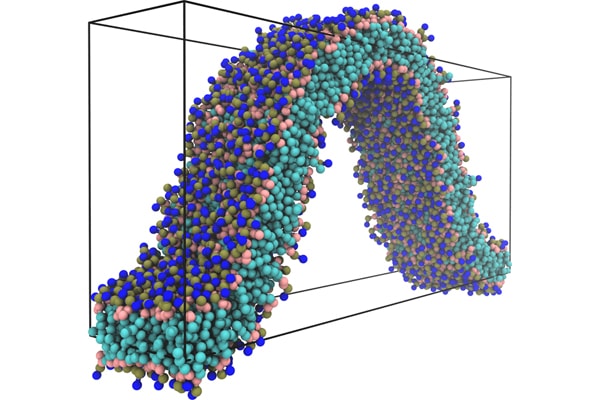
Markus Deserno uses theoretical and computational approaches – continuum elasticity theory and field theory as well as coarse-grained simulations – to study molecular-scale and mesoscale phenomena in biophysics. This allows him to study larger systems on longer time scales than in atomistic simulations and access a new arena for physical questions, many of which have biological significance. Specifically, Deserno investigates lipid membranes, proteins, viruses, or DNA on length scales larger than atomic resolution but smaller than a typical cell. On these scales, many fundamental physical concepts make a big impact on biology – among them thermal fluctuations, cooperativity, self-assembly, or elasticity. For instance, due to their surfactant-like nature individual lipid molecules in an aqueous environment spontaneously aggregate into membranes, which are laterally many orders of magnitude larger than their thickness. These quasi-two-dimensional fluid surfaces resist bending, a continuum elastic concept, but since the associated moduli are only about one order of magnitude bigger than thermal energy, membranes exhibit large thermal undulations that affect their properties.
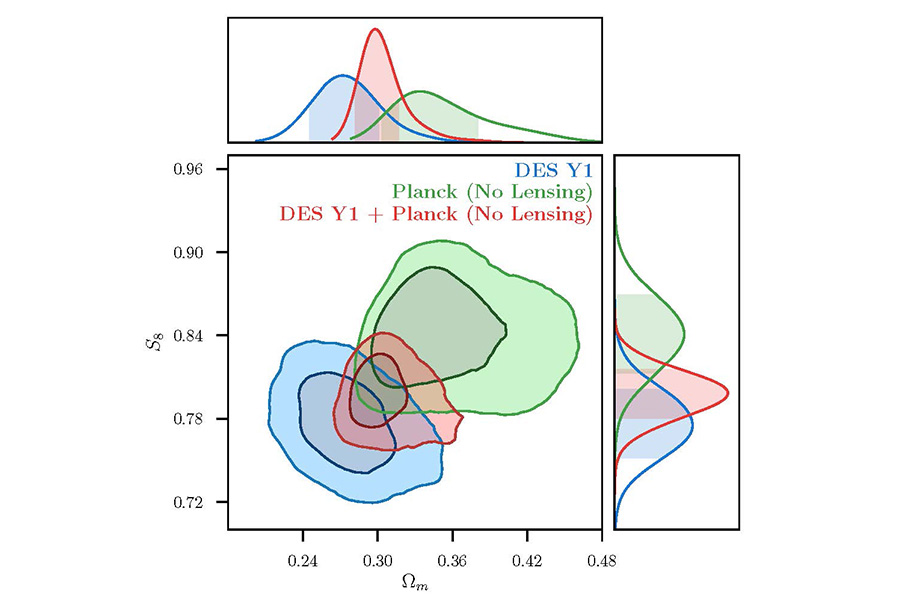
Scott Dodelson is interested in learning about fundamental physics by analyzing data from cosmic surveys. Astrophysicists have pieced together a remarkably successful cosmological model, but it requires three new pieces of physics: dark matter, dark energy, and inflation. His perception of the goal of cosmology over the coming decade is to extract as much information as possible from increasingly sensitive surveys to either learn about this new physics: What is the dark matter? Is the dark energy vacuum energy? If so, why does it have such a peculiar value? Did inflation really happen? If so, is there any way to relate the fields that drove inflation to those we know about today?
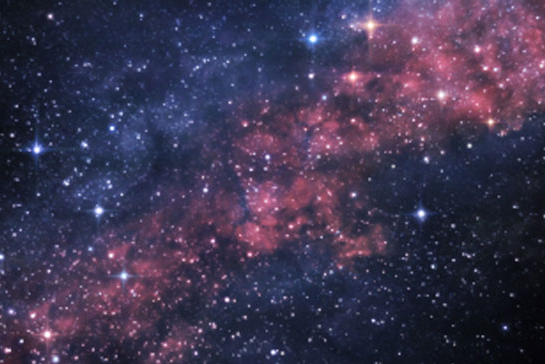
Tina Kahniashvili's research areas include investigation of physical processes in the Universe at very early epochs, as well as late times. In particular, she studies (i) fundamental symmetries tests at very high energies (early epochs of the universe expansion) using currently available data of astrophysical, cosmological, and particle physics experiments; (ii) gravitational waves signal from very early universe (inflation, phase transitions); (iii) CMB fluctuations in beyond standard cosmological models. She is interested in alternative scenarios to explain the accelerated expansion of the universe, such as modifications of general relativity (especially massive gravity models). She also works on cosmological magnetic fields and the origins, evolution, and observable signatures of primordial turbulence.
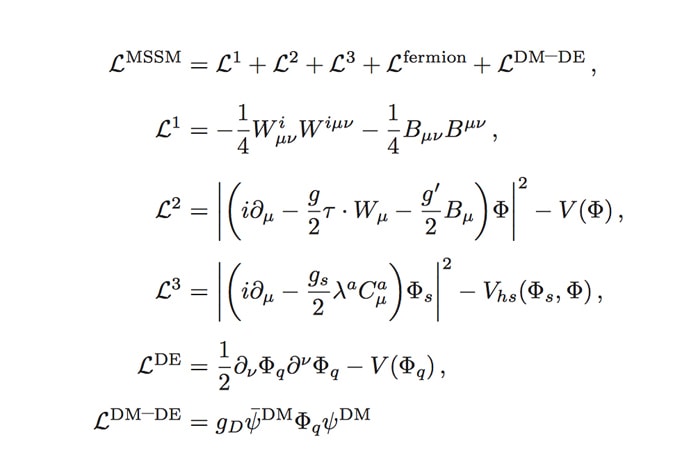
Leonard Kisslinger (Emeritus) uses a variety of techniques, such as QCD sum rules, Dyson-Schwinger equations, and light-front field theory, to study QCD and electroweak processes for deducing the structure of hadrons and nuclei, early universe phase transitions, cosmic electroweak bubbles, and pulsar kicks.
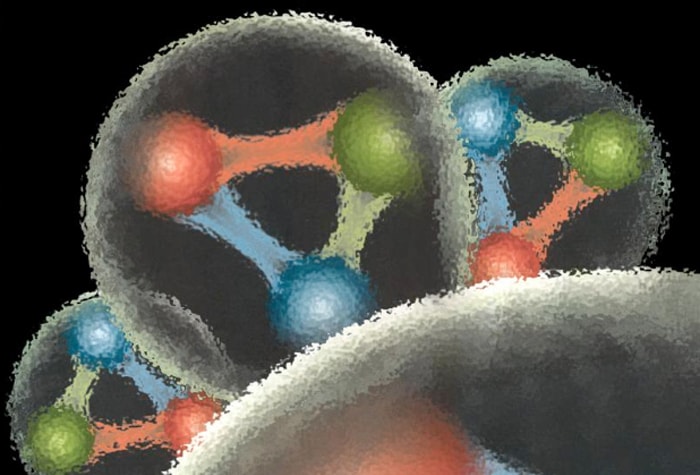
Colin Morningstar uses Markov-chain Monte Carlo computations in quantum chromodynamics (QCD) with a space-time lattice regulator to investigate hadron formation and quark confinement. He has computed the mass spectrum of glueballs in the Yang-Mills theory of gluons, studied the excitation spectrum of the effective QCD string between a static quark-antiquark pair, and obtained the first glimpse of the nucleon excitation mass spectrum from QCD. He is a member of a large nationwide collaboration of lattice QCD theorists dedicated to Monte Carlo calculations of QCD observables on large-scale computing clusters. He and Curtis Meyer built and maintain the CMU QCD cluster.
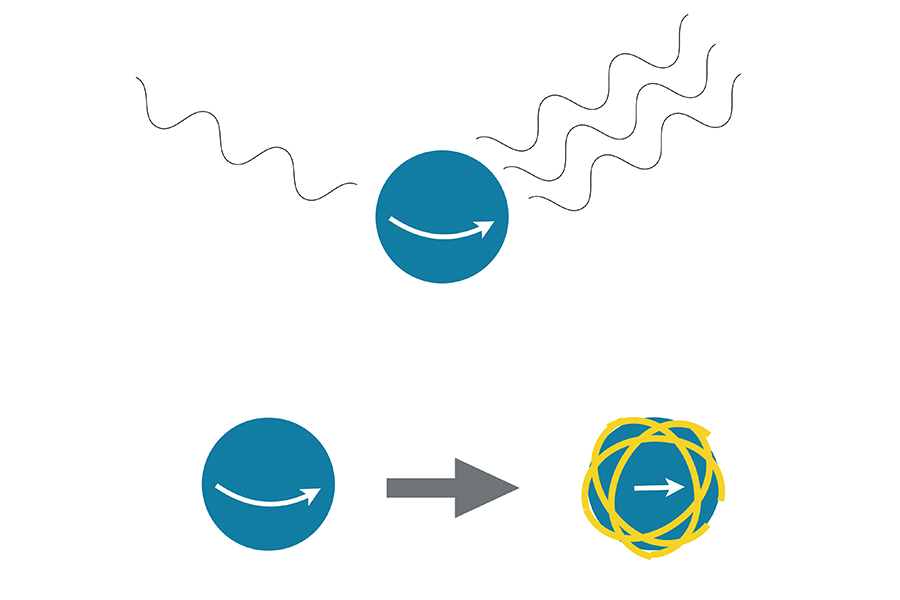
Riccardo Penco uses effective field theory (EFT) techniques to study a variety of phenomena in gravitational, high energy, and condensed matter physics. In the context of gravitational physics he has been studying on formal and phenomenological aspects of black hole physics. In high energy physics, he has been interested in exploring the relation between scattering amplitudes of different quantum field theories, and understanding their behavior at low energies. Finally, within the realm of condensed matter physics he has been focusing his attention on the dynamics and (self-)interactions of collective excitations.
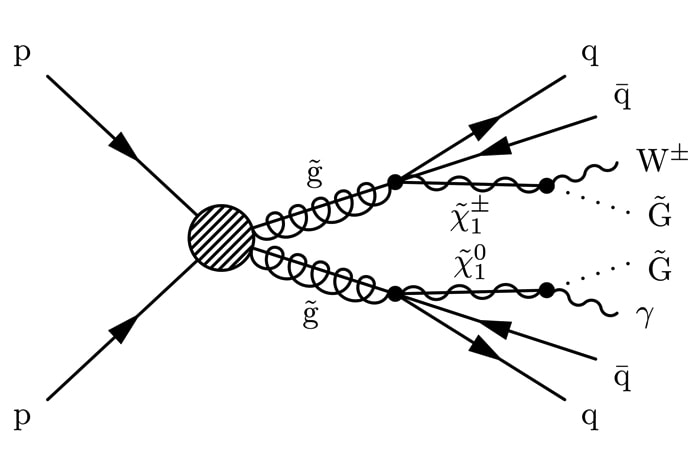
Ira Rothstein uses the data from the LHC to explain the origin of mass and the nature of the dark matter. He has worked on various topics in this field ranging from theories of extra dimensions to calculating Higgs boson production rates. He has also used quantum field theory to calculate classical gravity wave profiles for inspiralling black holes. He also works on effective field theory techniques to find systematic ways of calculating strong interaction observables at high energies.
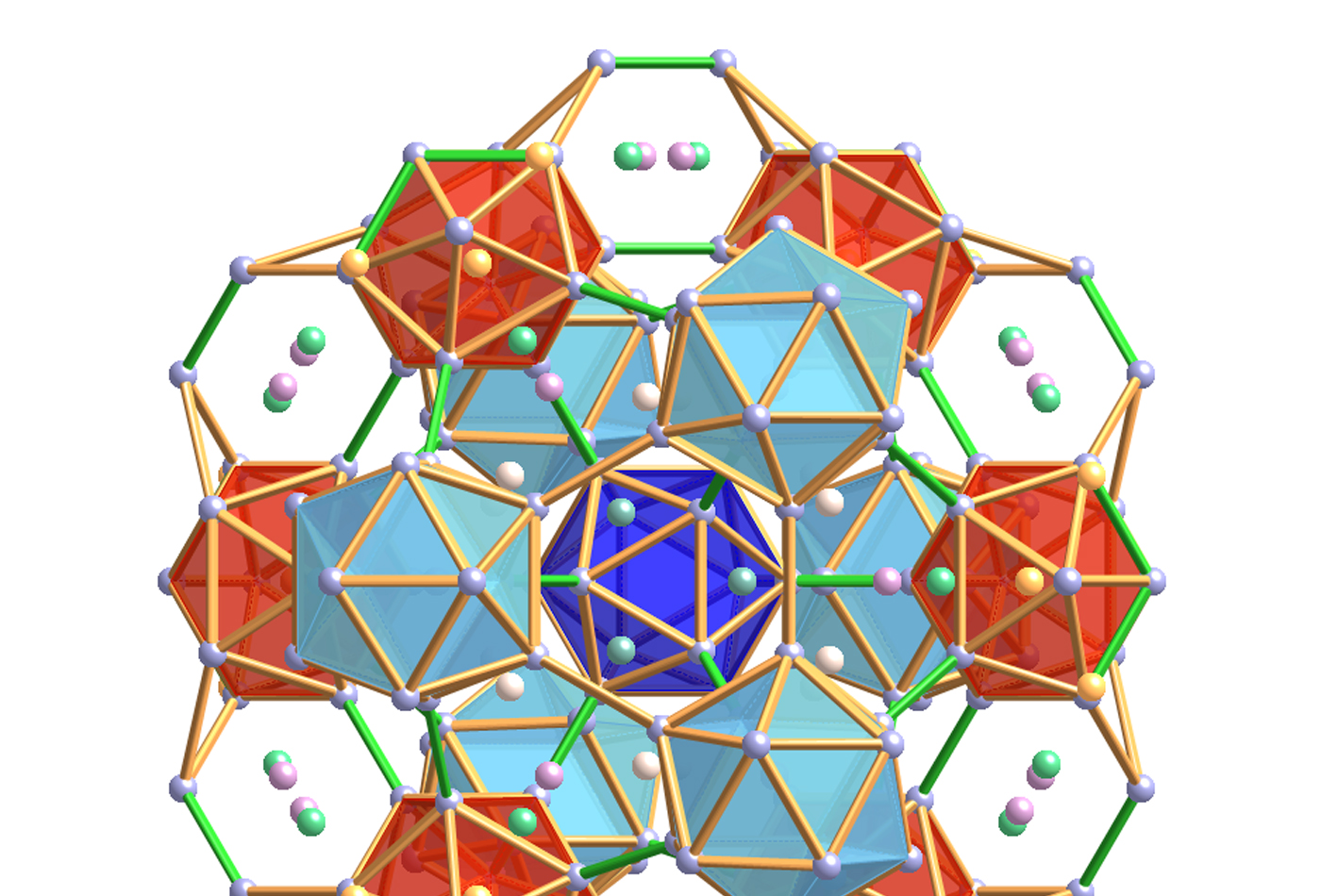
Michael Widom combines quantum mechanics-based total energy calculations with principles of statistical mechanics to model the structure and thermodynamic stability of complex metallic structures. Specific materials of current interest include liquid and amorphous metals, and structures with local or global icosahedral symmetry.