Friday, March 21, 2014
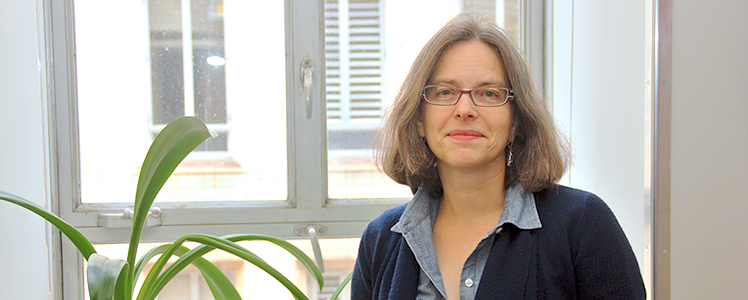
Computational Biologist Dannie Durand is Recreating Ancient History
Nearly three billion years ago, a group of bacteria thrived in the Earth’s primitive oceans. They fed on chemicals in the water around them, adapting to different conditions and resources. And when times got tough—the water was crowded with tons of other bacteria and resources were meager—they went into sleep mode by forming a spore, a tough structure that some bacteria use to survive during times of stress. Today these bacteria’s progeny are alive and well—and responsible for illnesses such as tetanus, botulism and anthrax.
These modern-day descendants of that long-ago ancestor have evolved different ways to decide when to make spores. How and why did that change happen?
Dannie Durand, a computational biologist at Carnegie Mellon University, is searching for answers, and she is traveling back through evolutionary time to do it. Durand is combing through the genomes of two classes of 21st century bacteria—the Clostridia and the Bacilli—to pinpoint the genes that are involved in their spore-making machinery. Using sophisticated sequence analysis and phylogenetic tools, she will create phylogenetic trees that trace the evolutionary trajectory of the bacteria and their spore-making machinery and then reconstruct what their ancestor’s spore-making proteins might have looked like.
“Our approach allows us not only to reconstruct what happened, but to ask how and why it happened the way it did,” said Durand, associate professor in the Departments of Biological Sciences and Computer Science, who is working on the project with John Pinney at Imperial College London and Michael Laub at MIT. The team recently received a Human Frontiers Science Program grant to carry out their work.
Similar to phylogenetic trees that show how cats, leopards and wolves are related, Durand’s bacterial family trees will look at how several classes of bacteria are related. But these trees will be highly specific—they will only deal with a particular set of genes involved in the cell signaling pathway that instructs a bacterial cell to make a spore.
Cells contain a myriad of signaling pathways that enable them to process information essential to their survival, such as sensing what is in their environment so that they can respond to sudden changes. If, say, a Bacillus receives a signal from its environment that indicates a lack of food, specific proteins will pass that message into the cell via a series of biochemical reactions, like a relay team that passes a baton from person to person. The message? Make a spore.
Clostridia and Bacilli, which descended from a common ancestor, both make spores and have very similar spore-making machinery. But the signaling pathways that tell the bacteria when to make a spore are different—the Clostridia have a simple, two-protein pathway while the Bacilli have a more complex four-protein pathway.
“Clostridia and Bacilli are the only classes of bacteria that make spores, which suggests that their common ancestor also made spores,” Durand said. “What did the ancestral pathway look like? Did it have two proteins or four? And how did the present-day pathways evolve from this ancestral pathway?”
To begin to answer these questions, Durand is characterizing the protein components of the modern day bacteria’s cell signaling pathways using sophisticated protein sequence analysis tools. With that information in hand, she’ll use a probabilistic method to infer what the ancient protein sequences might have been.
Predicting what the components of the ancient cell signaling pathway looked like is only one piece of the puzzle. Each component does not exist in isolation. They are wired together, like a circuit, to send and receive signals to relay the ‘make-a-spore’ command. Pinney, a Royal Society University Research Fellow, will determine how the components interacted with one another. With each step into the past, he will reconstruct how the ancestral cell signaling pathway components were wired and rewired over the course of more than two and a half billion years.
Durand and Pinney’s predictions of the ancient signaling pathway will give Laub, the Whitehead Career Development Associate Professor of Biology, the information he needs to reverse engineer the ancient sequences to bring it all back to life in the lab.
“Not only can we predict what the ancestor looked like, but we can make it,” Durand said.
Using the predicted protein sequences, Laub can make the ancient proteins and test their functionality with a series of experiments.
“What we see in today’s proteins is what works. Nature is implicitly telling us what doesn’t work,” Durand said. “Understanding evolution provides us with an enormous amount of information about how we could manipulate cells ourselves for medical and agricultural applications, including bioremediation and biofuel development.”