
Singled Out
MCS Biologists Examine Individual Neurons To Understand the Inner-Workings of the Brain
by Jocelyn Duffy
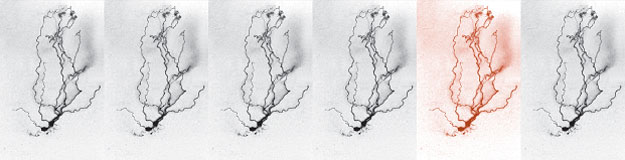
The brain, they say, is the body’s computer. Neurons are just transistors and circuits, relaying information. But our brains are far superior to a computer in many ways.
Take IBM’s Watson. It’s one of the smartest computers, at least when it comes to Jeopardy-style trivia. Watson can “think” and beat exceedingly smart humans, like Ken Jennings, at Jeopardy.
But if Jennings challenged Watson to a game of tic-tac-toe, Jennings would win without a doubt. Watson wouldn’t be able to place a single X or O on the grid without years of reprogramming. Computers like Watson can be exceptional at a few specific tasks, but they can’t perform the vast assortment of jobs that the brain can.
“There are a whole variety of tasks that humans and animals do effortlessly that we can’t figure out how to make a machine do,” said Nathan Urban, the Dr. Frederick A. Schwertz Distinguished Professor of Life Sciences and head of the department of biological sciences. “These types of tasks are what we need to be studying in order to better understand the brain.”
Take the sense of smell, for example. The brain’s olfactory system is made up of neurons that tell us when something smells like a flower or like a skunk. No computer can be made to identify and remember even the simplest odors, which, Urban says, is precisely why such things need to be studied.
Seeing the Beauty of Diversity
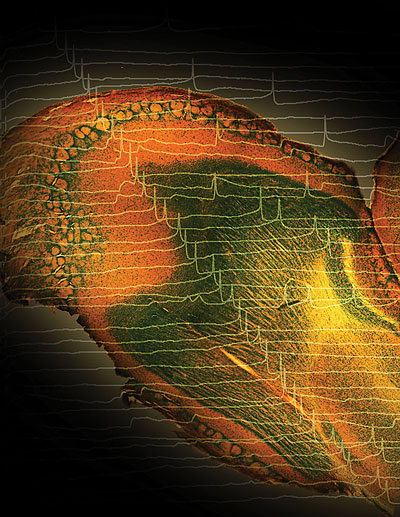
Artist’s rendering of an olfactory neuron, layered with recordings of neuronal activity. By Sonya Giridhar, CNBC graduate student working in Nathan Urban’s laboratory.
Our brains are made up of 100 billion cells, give or take. These cells, called neurons, fire in connection with one another to make us smell, see, hear, feel, think and learn. If the brain was like a computer, all of its neurons would be mass-manufactured circuits, each one exactly the same as the next. But that isn’t the case.
“When we think about computer chips, variability in hardware clearly can be very destructive. Manufacturers spend a lot of time and expense making sure each transistor or memory element on a chip is identical,” Urban said. “The brain is considered to be one of the most sophisticated computers there is, but neurons are nowhere near being identical.”
Neurons can vary in shape, size, the number of connections they make and the kinds of chemical signals they send. They can also vary in the way they fire electrical impulses.
Since neuroscientists first began to record neuronal activity, they observed that individual cells behaved differently, firing at different rates and intensities. They assumed that this was merely a flaw of biology and averaged the differences away when analyzing their data.
While studying how neurons in the olfactory system communicate to detect smell, Urban’s postdoctoral associate Krishnan Padmanabhan noticed that in response to the same stimulus, each neuron fired at its own unique rate and pattern. Urban’s initial response was that the results were normal. But then he began to wonder whether such variability was truly the flaw that everyone thought it was. In further experiments, Urban and Padmanabhan found that it was no flaw. Each neuron coded a subtle yet different piece of information about the stimulus. The differences in firing were the equivalent of transmitting a message in 3-D, high definition and surround sound. When Urban made the neurons fire in the exact same way, they coded less diverse information, delivering a message in low resolution, black and white.
These differences, Urban says, illustrate why it’s essential to study the brain at the level of the individual neuron. MCS biologists are at the forefront of this field of study. Their work stands to fundamentally change how we understand the brain and the role it plays in learning, memory, health and disease.
Finding the Neuron in the Haystack
Neuroscientists have made great strides in identifying the general areas of the brain that perform certain tasks, such as seeing or producing language. But these methods give a broad, low-resolution picture that doesn’t show individual neurons. Until recently, individual neurons could only be studied ex vivo, by examining slices of brain under a microscope. MCS biologists are among scientists developing new methods that are changing this, allowing scientists to see and track neurons in vivo.
“Non-invasive imaging has played an important role in the advancement of neuroscience over the last 25 years,” said Eric Ahrens, associate professor of biological sciences. “Using non-invasive methods, we can now see inside the brain. This has been huge for the field.”
For decades, physicians and scientists have used imaging techniques like MRI to peer inside living brains for diagnostic purposes, seeing larger structures like tumors or lesions. Ahrens has developed a way to use MRI to visualize the much smaller neuronal stem cells.
Contrary to popular belief, the brain is constantly producing new neurons. These neural stem cells, called neuroblasts, are born deep in the brain. As they travel through the nervous system, they mature into neurons. Ahrens tags the neuroblasts with a gene for an engineered ferritin, a nature-inspired protein that attracts iron. The iron turns the cells into nanomagnets, enabling them to be viewed using MRI. By watching the cells as they migrate through the brain, Ahrens believes we’ll get a better idea of the molecular cues the neuroblasts follow to get to their final destination. Ideally, these cues could then be manipulated to redirect the neuroblasts to injured areas of the brain, delivering a source of regenerated cells.
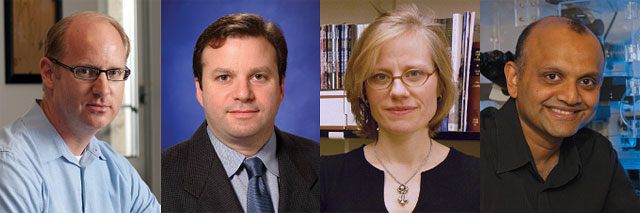
From left: Nathan Urban, Eric Ahrens, Alison Barth and Manoj Puthenveedu.
Imaging can also be used to show when a neuron is firing an electrical impulse. Several years ago, Alison Barth, associate professor of biological sciences, developed a novel tool that can be used to visualize, in living brain tissue, a single neuron that has been activated in response to an experience. She created a transgenic mouse that couples green fluorescent protein (GFP) to a gene activated by neuronal firing, causing the neuron to glow when it fires. Researchers can use this glow to see which neurons react to a given stimulus and how they connect with one another when the brain works to complete a task. For example, when a mouse learns how to solve a maze, Barth can visualize what neurons it used, and later study those neurons to find out how they changed to facilitate learning.
“Typically we studied neurons based on what type of neuron they were or where they were located,” said Barth. “Now we can study them based on what they do. It’s a new and exciting way to study the brain.
Peering Inside the Neuron
Researchers around the world are using Barth’s transgenic mice to study an array of brain activities. The models often are used to find out which neurons are active in different neurological diseases.
Barth has used her model to isolate neurons that are activated during a seizure. Once she located the neurons of interest, Barth examined each neuron and found a disruption in an ion channel called the BK channel. This disruption caused neurons to become overly excitable, which contributed to recurrent seizures— the hallmark of epilepsy. Barth has since studied the channels as a pharmaceutical target for epilepsy, and continues to look at neurons to see how they contribute to the seizure disorder.
In another MCS lab, Assistant Professor of Biological Sciences Manoj Puthenveedu is using a different imaging tool to look at the opioid receptors at the surface of a neuron’s cell membrane. In doing so, he hopes to find clues to the mechanisms that contribute to addiction.
Under normal circumstances, like during a strenuous jog, opioid receptors recognize endorphins and activate specific signals in the brain’s cells to counter pain and discomfort. These same receptors also recognize drugs like heroin and morphine, and activate the same exact signals.
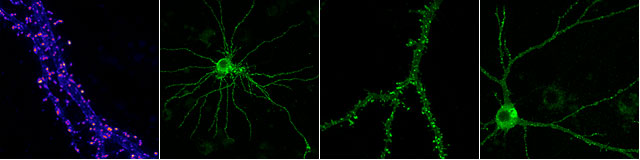
From the lab of Manoj Puthenveedu, a neurotransmitter receptor tagged with green fluorescent protein and expressed in cultured neurons.
“There is no difference in the cellular signals that are activated by drugs and endorphins in the brain. But we don’t get addicted to endorphins that easily. Why?” said Puthenveedu.
In an attempt to find out, Puthenveedu uses live-cell confocal fluorescence microscopy to watch opioid receptors as they move in and out of the cell in response to opioids. He can see differences in what happens when the receptor recognizes a drug and when it recognizes an endorphin. By analyzing these differences, he hopes to identify what changes in the molecular pathway cause the brain to become addicted.
Looking at the Big Picture
Ultimately, to fully understand the brain, the information that is taken at the level of the single neuron needs to be put into a more global context. Researchers need to know how the individual neurons connect and communicate with other neurons. Urban is doing that, interestingly enough, with a computer.
“The brain has 100 billion cells. If we’re going to understand the collective behavior of those cells, the most useful approach is to develop a computer simulation,” said Urban.
Urban has taken information about single neurons gathered using experimental approaches to build a model of the behavior of a single neuron. Then, based on that model, he is able to compute how a large number of neurons would behave given a particular stimulus.
In all, the techniques MCS biologists are using—whether they’re computer simulations focused on large groups of brain cells or studies of the tiny mechanisms within a single neuron— stand to provide a better understanding of how the neurons in our brains connect and communicate to make the brain the world’s most sophisticated computer.
Brain, Mind & Learning at Carnegie Mellon
In January 2011, CMU launched the Brain, Mind & Learning Initiative, which brings together and supports researchers from diverse disciplines including biology, psychology, computer science, statistics and bioengineering as they work to understand and harness the power of the brain.
“Carnegie Mellon is the perfect place to do this type of research. Computer scientists are studying computational processes behind learning and psychologists are studying cognitive processes. The biologists across campus are studying the underlying biological processes. It makes sense to pull it all together,” said Nathan Urban.
To learn more, visit Brain, Mind & Learning.