|
|
|
Tethered Bilayer Lipid Membranes (tBLMs)
Duncan J. McGillivray,§,†,‡ Frank Heinrich,§,† Gintaras Valincius,* David J. Vanderah,† Mathias Lösche§,†
§Carnegie Mellon University, Pittsburgh, PA; †National Institute of Standards and Technology, Gaithersburg, MD; *Institute of Biochemistry, Vilnius, Lithuania
‡Now at the Dept. of Chemistry, The University of Auckland, New Zealand
Protein-Membrane Interactions in Cell Biology
The interaction of proteins with membranes is of tremendous importance for many cellular processes. Seemingly minor changes in the interaction of a protein with the biomembrane may result in severe pathologies, such as the premature aging disease, Hutchinson-Gilford Progeria Syndrome (HGPS). In HGPS, a single point mutation in LMNA, the gene encoding the membrane-associated protein Lamin A, leads to a Lamin A variant that shows an abnormally high affinity to the inner surface of nuclear membrane – the location where a protein network, the nuclear lamina, forms a support structure which helps define the shape of the nucleus. As a result, the mechanical properties of the nuclear membrane as well as the morphology of the nucleus are drastically altered.
And much worse: Complex mechanisms that are poorly understood to date lead to premature aging of the affected individuals starting from earliest childhood. Limited growth, hair loss, osteoporosis, atherosclerosis and cardiovascular problems leave these kids with a phenotype of advanced senescence. Affected patients usually die before they reach age 20.
How can such a small difference in membrane-protein interaction yield such devastating results? – To provide answers to such questions, we clearly need to develop experimental systems that permit investigations of the structure, function, dynamics and kinetics of protein association with membranes in molecular detail! One particular challenge is that cell membranes are intrinsically highly disordered systems that rely on this disorder for its physiological functioning, and are thus is not well suited to crystallography studies. Furthermore, only a small number of membrane-integral proteins have been crystallized – and this number is growing much more slowly than for soluble proteins [1]. Solid support allows membranes to be investigated by AFM, ellipsometry, neutron reflectometry (NR), and electrochemical methods including electrical impedance spectroscopy (EIS). These membranes are then a more biologically relevant surrounding in which proteins can be investigated to obtain structural and functional clues. The solid support also makes systems robust, which enables the development of biosensor applications.
|
A powerful experimental system: The tBLM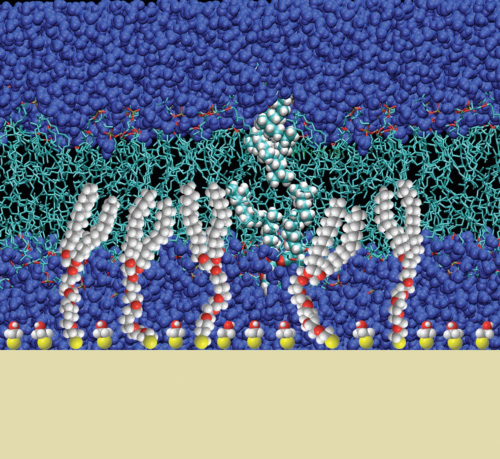
Our recent work has focused on the optimization and characterization of model systems for the reconstitution of pore-forming membrane proteins. We have designed a bilayer membrane system, which is tethered to a gold-substrate and contains a nanometer-thin solvent reservoir between the membrane and the substrate. Such systems, termed tethered bilayer lipid membranes (tBLMs), are now beginning to be useful to probe membrane-active proteins and their interaction with the bilayer.
The tBLMs developed in this work are based on thiolipids synthesized at NIST, in which the two alkyl tails are joined though a glycerol to a thiolated oligo(ethylene oxide) “spacer”. This spacer supports the membrane above a gold surface, providing the potential for a sub-membrane solvent reservoir. Such a reservoir is necessary to enable reconstituted transmembrane proteins to extend beyond the membrane, and helps to reduce the effects of the solid surface on the membrane. NR studies on the AND/R instrument at the NIST Center for Neutron Research, supported by EIS, show that complete, defect-free membranes can be formed on these tethers using an adaptation of the “rapid solvent exchange” method developed by Cornell et al. [2]. But NR measurements also showed early on that these membranes did not contain a solvent reservoir, implying the oligo(ethylene oxide) spacer is filling the sub-membrane space.
To design a hydrated submembrane reservoir, thiolipids are spaced out on the surface by using β-mercaptoethanol (βME) for the formation of a self-assembled monolayer (SAM) in the first preparation step. This method decreases the amount of the tether on the surface, and NR shows that the resulting tBLMs do indeed comprise aqueous solvent between the bilayer membrane and the substrate. Moreover, fluorescence correlation spectroscopy (FCS) shows that the diffusion constant of a lipid-bound label is indistinguishable from that measured in free-standing bilayers, such as those forming giant unilamellar vesicles.
|
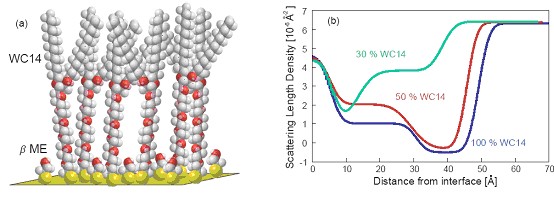
(a) Illustration of a mixed SAM on gold, consisting of the PEGylated thiolipid, WC14, which is diluted on the surface by βME molecules. (b) NR scattering length density profiles show the decreasing amount of WC14 on the surface as its proportion in the SAM-forming solution decreases, with the collapse of the WC14 in sparsely-tethered systems.
|
It is for these “sparsely-tethered” membrane systems that the rapid-solvent exchange technique is most useful, as vesicle-rupture techniques do not work well on these modestly hydrophilic SAMs. However, solvent exchange with a variety of phospholipids produces tBLMs that are electrically sealing, as EIS shows, with low capacitance and high resistivity. Importantly, we establish unmistakably from NR measurements a distinct hydrated reservoir of about 20 Å under a sparsely-tethered membrane [3,4]. |
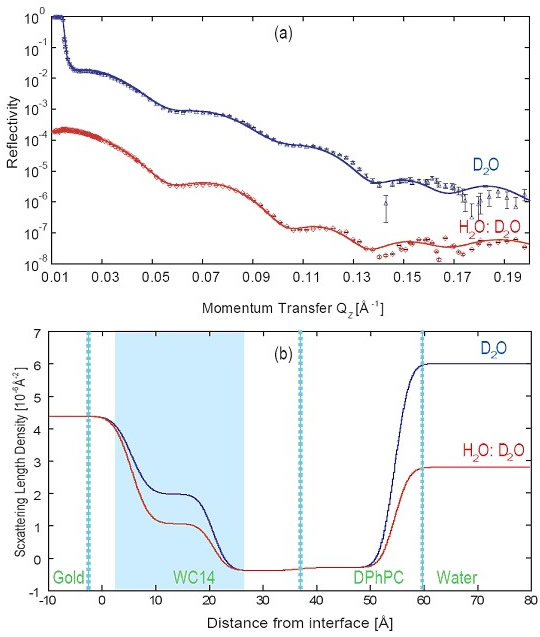
(a) Example of early NR measurements Duncan performed at the NCNR at two distinct solvent contrasts on a tBLM from a 1:1 mixture of WC14 and βME with DPhyPC as the fill lipid.
(b) The nSLD profiles show that the change in isotopic solvent composition does not only affect the scattering power in the semi-infinite buffer over the bilayer (at distances > 60 Å from the gold surface) but also in the submembrane space (shaded in blue). This is an indication that the narrow cleft between the membrane and the substrate is hydrated by material transport across the bilayer, presumably through defects.
|
Phospholipase: An Amplifier of Local Defect Structure
tBLMs have been further characterized by challenging with the phospholipase PLA2. This protein cleaves the acylester bond in the sn-2 position of glycerophospholipids, thereby destabilizing lipid membranes. PLA2 activity is known to depend on the short-range intrinsic order of the membrane, and can therefore be used to probe the fluidity and the nature of defects in the lipid layer [5]. tBLMs prepared under otherwise identical conditions from diphytanoyl-phosphatidylcholine (DPhyPC) and from palmitoyl-oleoyl-phosphatidylcholine (POPC) were investigated.
Without the cofactor Ca2+, both tBLMs are stable in the presence of PLA2. However, Ca2+ addition triggers enzyme activity and leads to rapid degradation of the POPC bilayer while the DPhyPC bilayer remains intact. These results show that tBLMs from different constituents, which are quite similar by electrochemical and structural characterization, can be vastly different in their sensitivities to PLA2 and, by inference, also in local structural detail [6]. |
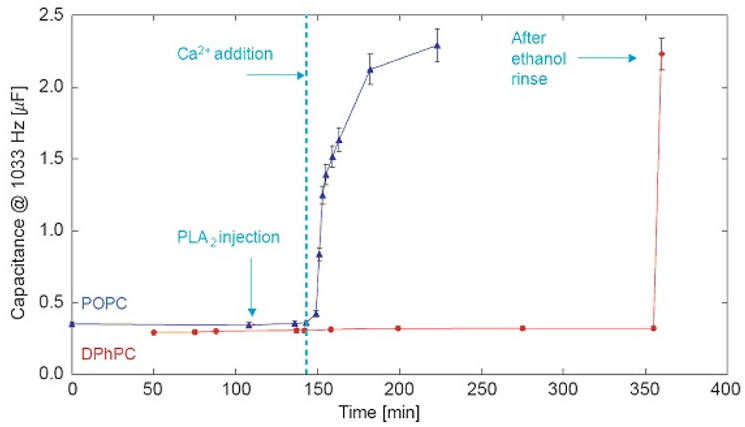
EIS shows the Ca2+-triggered response of tBLMs (DOPC or DPhyPC on 7:3 βME:WC14) to PLA2.
|
Ongoing Research
Similarly, variation of structural details of the thiolipid anchor lead to measurable differences in tBLM properties. Two related anchor compounds which we currently use, designed by Dave Vanderah and designated as WC14 and FC16, differ in their ability to form tBLMs at high dilution with βME [4]. FC16, which has longer tails – palmityl vs. myristyl – than WC4 and a slightly longer hydrophilic spacer – nona(ethyleneoxide) vs. hexa(ethyleneoxide), is capable of forming tBLMs if diluted with βME up to 15:85 [4] while for WC14, the optimum is 30:70 [3]. On the other hand, tBLMs based upon FC16 have consistently a slightly smaller diffusion constant than those based upon WC14, as determined by Sidd Shenoy in our lab in z-scan FCS measurements. This is presumably due to the slight increase in Van-der-Waals interactions between the aliphatic chains, which are slightly longer in FC16. Curiously enough, these dynamic properties encroach upon the bilayer leaflet distal to the gold surface, i.e., the one that does not incorporate the lipid anchor.
|
This research has been published in a series of publications, including refs. [3], [4] and [6] below. It was supported by the National Science Foundation (grants #0555201 and 0457148) and is continued under the sponsorship of the Department of Commerce MSE program (70NANB8H8009) and the American Health Assistance Foundation (AHAF, grant #A2008-307). This project benefits greatly from neutron neutron research facilities provided by the National Institute of Standards and Technology at the NIST Center for Neutron Research.
References
[1] White, S. H. (2004), Protein Science 13, 1948.
[2] Cornell, B. A.; Braach-Maksvytis, V. L. B.; King, L. G.; Osman, P. D. J.; Raguse, B.; Wieczorek, L.; Pace, R. J. (1997), Nature 387, 580.
[3] McGillivray, D. J.; Valincius, G.; Vanderah D. J.; Febo-Ayala, W.; Woodward, J. T.; Heinrich, F.; Kasianowicz, J. J.; Lösche, M. (2007), Biointerphases 2, 21.
[4] Heinrich F.; Ng, T.; Vanderah, D. J.; Shekhar, P.; Mihailescu, M.; Nanda, H.; Lösche, M. (2009), Langmuir 25, in press.
[5] Jørgensen, K.; Davidsen, J.; Mouritsen, O. G. (2002), FEBS Letters 531, 23.
[6] Valincius, G.; McGillivray, D. J.; Febo-Ayala, W; Vanderah, D. J.; Kasianowicz, J. J.; Lösche, M. (2006), J. Phys. Chem. B 110, 10213. |
|
|
|