Matteo Cremonesi
Assistant Professor
Wean Hall
Nuclear and Particle PhysicsHigh Energy Physics Experiment
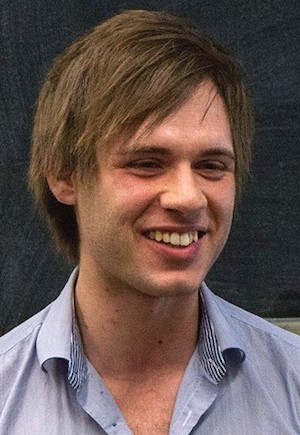
Education & Professional Experience
M.S.:University of Rome II (2012)
B.S.:University of Rome II (2009)
LHC Physics Center Artificial Intelligence Fellow & Research Associate at University of Notre Dame, 2021-
Research Associate at Fermi National Accelerator Laboratory (FNAL), 2015–2021
Fellow at Harvard University Department of Physics,, 2013
Research Interests
As an experimental particle physicist, my goal is to study the fundamental building blocks of Nature. The standard model (SM) of particle physics, the theory that describes the forces that regulate the interaction between the elementary particles, has provided a very successful description of the Universe, validated by decades of experiments. As a PhD student I contributed to one of these validations. As a member of the Collider Detector at Fermilab (CDF) experiment, that operated at the Tevatron collider at Fermi National Accelerator Laboratory in Chicago, I had the opportunity to co-lead the effort that discovered one of the predicted but at the time still unobserved production mechanisms of the top quark, the heaviest of all elementary particles. Despite the success of the SM, many questions at the forefront of particle physics remain unanswered, one of the most important being the nature of dark matter (DM). Experiments at the Large Hadron Collider (LHC) at CERN in Geneva, are primarily positioned to address some of these questions.
Although cosmological observations demonstrate that around 85% of the mass of the Universe is comprised of DM, details of its composition remain elusive. If DM particles interact with SM particles, DM can be produced by colliding protons at high energy. While the LHC is at the energy frontier of colliding beams, its experiments were not designed to directly detect DM: once produced, DM particles would not interact with the detectors, passing through them without leaving any trace. However, if they are produced in association with other detectable particles, they can manifest themselves as an imbalance in the total momentum. The estimate of the momentum that has been carried away by non detectable, “invisible” particles, but that is expected due to the law of conservation of energy is called missing transverse momentum (MET). At the LHC, an entire program has been built on searches for DM in collisions that produced large MET. As a member of the Compact Muon Solenoid (CMS) experiment, I contributed to this program publishing two searches for DM produced in association with a top quark and in association with a SM Higgs boson.
I was also selected as the CMS delegate to the LHC DM Working Group, a cross-experiment effort that defines a set of shared guidelines and recommendations for the LHC physicists on interpretation, presentation, and characterization of DM results.
The LHC DM program on searches with large MET is currently limited by the performance of the MET reconstruction. To improve the MET that is used for the offline analysis of the data collected by CMS, I am leading the development of a graph neural network (GNN) for MET reconstruction. My work took inspiration from existing machine learning (ML) MET reconstruction methods and showed a significant and promising improvement. From 2020 to 2022 I also led the MET group of the CMS experiment, where I promoted the adoption of ML techniques while simultaneously maintaining and improving the more classical MET reconstruction approaches. Although a crucial aspect, the resolution of the MET used for offline analysis is not the only bottleneck for MET-based DM searches. At the LHC, millions of collisions are delivered every second, but only a fraction of those can be stored by the experiments, due to the limited bandwidth, processing, and storage resources. In collider experiments, a mechanism called the "trigger" classifies and selects events in real-time. It is composed of a combination of algorithms, implemented in both hardware and software, that save only the events with characteristics of interest as they occur. The hardware component has only a few microseconds to decide whether to record events. Due to these extremely low latencies, the algorithms used at this stage are deployed on custom electronics, like field-programmable gate arrays (FPGAs). My plan is to develop tools for the deployment on FPGAs of GNNs for MET reconstruction similar to the one developed for offline analysis. Such tools will be rather general and could be applied to the deployment of GNNs developed for other scopes. Furthermore, while microsecond latency requirements are unique to CMS, the ability to perform real-time analysis with high efficiency could be relevant to other applications in physics and beyond. Real-world applications like on-device image processing performed by intelligent cameras or the navigation of autonomous vehicles may benefit from the same techniques.
Selected Publications
N. Smith et al., Coffea – Columnar Object Framework For E˙ective Analysis, EPJ Web of Conferences 245, 06012 (2020).
M. Cremonesi et al., Using Big Data Technologies for HEP Analysis, EPJ Web of Conferences 214, 06030 (2019).
A. M. Sirunyan et al., Search for dark matter propduced in association with a Higgs boson decaying to a pair of bottom quarks in proton-proton collisions at (s) = 13 TeV, Eur. Phys. J. C 79 (2019) 280.
A. M. Sirunyan et al., Search for darkpmatter in events with energetic, hadronically decaying top quarks and missing transverse momentum at (s) = 13 TeV, JHEP 06 (2018) 027.
O. Gutsche et al., CMS Analysis and Data Reduction with Apache Spark, JPCS 1085 042030 (2018).
O. Gutsche et al., Big Data in HEP: A comprehensive use case study, JPCS 898, 072012 (2017).
T. Aaltonen et al., Measurement of the single top quark production cross section and |Vtb| in 1.96 TeV pp¯ collisions with missing transverse energy and jets and final CDF combination, Phys. Rev. D 93, 032011 (2016).
T. Aaltonen et al., Tevatron Combination of Single-Top-Quark Cross Sections and Determination of the Magnitude of the Cabibbo-Kobayashi-Maskawa Matrix Element |Vtb|, Phys. Rev. Lett. 115, 152003 (2015).
T. Aaltonen et al., Search for Resonances Decaying to Top and Bottom Quarks with the CDF Experiment, Phys. Rev. Lett. 115, 061801 (2015).
T. Aaltonen et al., Constraints on Models of the Higgs Boson with Exotic Spin and Parity using Decays to Bottom-Antibottom Quarks in the Full CDF Data Set, Phys. Rev. Lett. 114, 141802 (2015).
T. Aaltonen et al., Observation of s-Channel Production of Single Top Quarks at the Tevatron, Phys. Rev. Lett. 112, 231803 (2014).
T. Aaltonen et al., Search for s-Channel Single-Top-Quark Production in Events with Missing Energy Plus Jets in pp¯ Collisions at ps = 1.96 TeV, Phys. Rev. Lett. 112, 231805 (2014).